On this page
It is not necessary to have an in-depth knowledge of the immune system to understand the first principles of vaccinology. The immune system is an extremely complex inter-connected system, but understanding certain aspects involved in the process of inducing specific immunity through vaccination inform vaccination practice.
As protective immunity develops over time, the timing of vaccine doses, along with a basic understanding of the different types of vaccines, becomes important.
1.1. Immunity and immunisation
Immunity is the biological state of being able to resist disease or a toxin: the primary objective of vaccination is to induce an immunological memory against specific diseases, so that if exposure to a disease-causing pathogen occurs, the immune response will neutralise the infection or toxins it releases before disease can occur.
1.1.1. Immune recognition
1.1.1. Immune recognition
One of the primary ways in which the immune system achieves elimination of pathogens and other unwanted foreign material is being able to distinguish ‘self’ from ‘non-self’. Each cell in the body is equipped with a type of molecule that identifies the individual from any other, much like a 3D barcode. Pathogens not only lack the individual’s ‘self’ marker, they also contain ‘virulence factors’ that alert the immune system to danger.
Antigens (antibody generators) are the drivers of the specific immune response. Antigens are molecular shapes, such as part of a protein or glycoprotein, that the immune system recognises as foreign and can trigger an adaptive immune response. While some vaccines contain the entire weakened or attenuated organism (live viral vaccines like measles, mumps and rubella vaccines), increasingly, newer vaccines contain purified or recombinant protein antigens (as in acellular pertussis, HPV or pneumococcal vaccines).
The first process that occurs when a foreign antigen, such as a vaccine antigen, is introduced to the body is the recognition that the antigen is non-self by triggering an inflammatory response. The antigen is taken up at the local site (such as the injection site) by specialist phagocytic cells called antigen-presenting cells – macrophages and dendritic cells. Once inside the antigen-presenting cells, the foreign protein (or microbe) is dismantled into tiny fragments that are displayed on cell surface alongside a ‘self’ molecule. These antigen-presenting cells carry the antigen to through the lymph to the local lymph node where the adaptive immune response is initiated.
1.1.2. Induction of the adaptive immune response
1.1.2. Induction of the adaptive immune response
The response that occurs the first time an antigen is ‘seen’ by the immune system is called the primary immune response.
The adaptive immune responses occur in lymphoid tissue, primarily in the spleen and in the 500–600 lymph nodes distributed throughout the body.
The adaptive immune response to most vaccines occurs at the draining lymph node proximal to the site of injection. The spleen and lymph nodes are densely populated with important effector lymphocytes of the immune response: T-cells and B-cells. In the lymph node, the vaccine antigen is presented to the specific T‑cells and B-cells.
Among the trillions of specific T and B lymphocytes (there are ~1016 possibilities), there usually exists a match for the antigen. Cells that recognise the antigen are activated through communication with the antigen presenting cell and the primary immune response can be initiated. This process and the response matures over a period of four to six weeks.
An early outcome of the interaction between these antigen-presenting cells and T and B lymphocytes is the production of antibody-producing B-cells. Antibody can be measured in the blood as soon as 4–7 days after this interaction, but is usually more effectively measured weeks to months later. Initially, this is low in quantity and of low affinity for the antigen (it binds weakly to the antigen), and primarily consists of the antibody subtype immunoglobulin M (IgM), often referred to as ‘early antibody’. It peaks at around 10 days then declines relatively quickly (see Figure 1.1).
For most vaccine-preventable diseases this process is too slow following infection, and disease occurs before an effective immune response can be mounted. Injecting a part or a weakened version of the pathogen in the form of a vaccine, readies the immune system so that it can mount a more rapid and effective response when the wild disease is encountered.
1.1.3. Development of immune memory and the secondary response
1.1.3. Development of immune memory and the secondary response
The response that occurs the second time an antigen is ‘seen’ by the immune system is called the secondary immune response.
During the primary immune response, over a period of around two months, cells that are less specific for the specific antigen are deleted, and those that are highly specific are retained and multiply within the lymph node. Antibody production also switches from IgM to more specific IgG or IgA subtypes. During this time immunological memory cells also develop, but it takes around four months, after the initial antigen is cleared, to fully form immune memory.
The next time the same antigen is introduced, either as a pathogen component or as a further dose of vaccine, the immunological memory cells that recognise it will be activated and begin to proliferate. Highly specific antibody (primarily of the IgG subtype, but also IgA) is rapidly produced in large amounts. The lag phase is much shorter than the primary immune response (see Figure 1.1), at just 1–4 days; the antibody level peaks very quickly and lasts much longer.
The immune system has been readied by the vaccine; if the actual disease pathogen enters the body, then it is recognised promptly and neutralised by the immune system preventing it from causing disease.
Figure 1.1: Comparison of primary and secondary immune responses to protein-containing vaccines
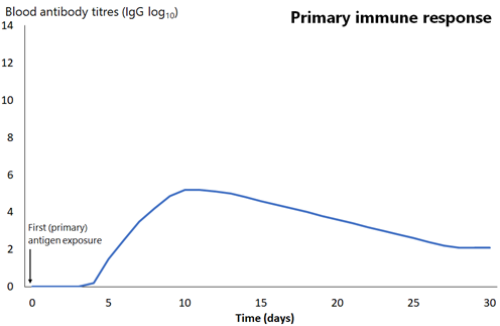
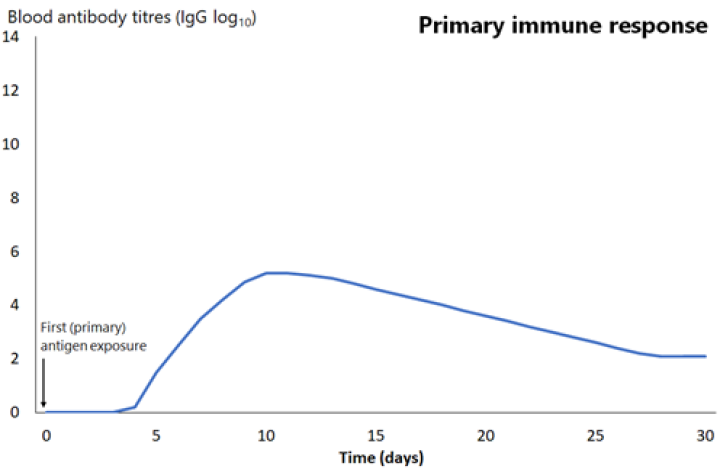
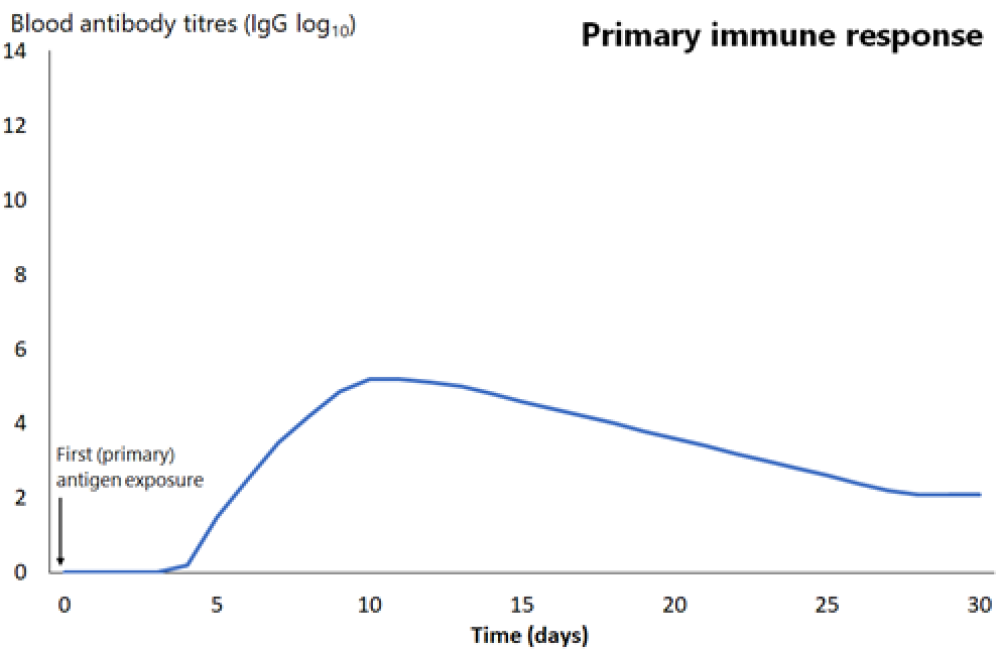
Secondary responses are faster (peaking at day 7) than the primary immune response and the antibody titres are higher, more prolonged and of higher neutralising capacity.
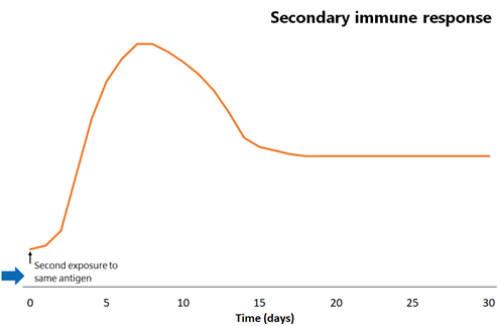
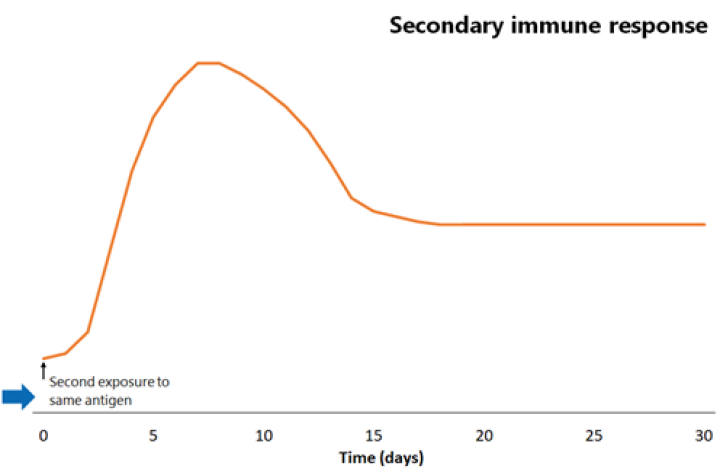
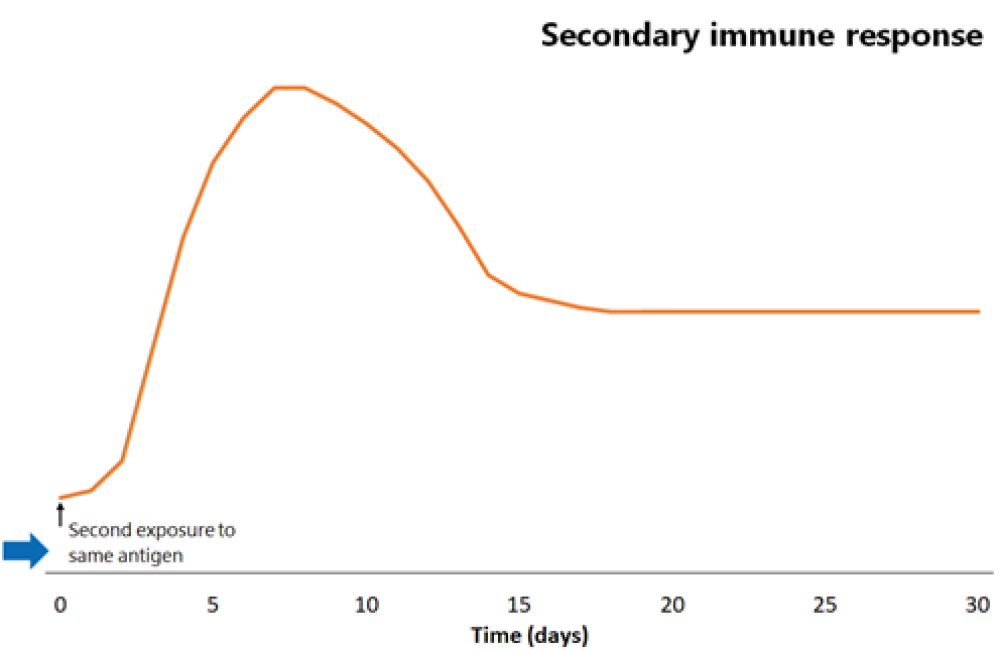
Secondary responses are faster (peaking at day 7) than the primary immune response and the antibody titres are higher, more prolonged and of higher neutralising capacity.
Secondary responses are faster (peaking at day 7) than the primary immune response and the antibody titres are higher, more prolonged and of higher neutralising capacity.
Innate immunity
Most infectious microbes (also known as micro-organisms) are prevented from entering the body by barriers such as skin, mucosa, cilia and a range of anti-microbial enzymes. Any microbes that breach these surface barriers are then attacked by other components of the innate immune system, such as polymorphonuclear leucocytes (neutrophils), macrophages and complement.
This innate immune response is a pre-programmed non-specific response that does not involve learnt or adaptive mechanisms. The cells and proteins of the innate immune system can recognise common microbial markers or virulence factors and can kill microbes without the need for prior exposure. The chemical messages (cytokines) and cells of the innate immune system also interact with the cells of the adaptive immune system (eg, lymphocytes) to induce a cascade of events that leads to adaptive, antigen-specific immunity and immune memory, as summarised in Figure 1.2.
Figure 1.2: Summary of non-specific innate and adaptive (specific) immunity
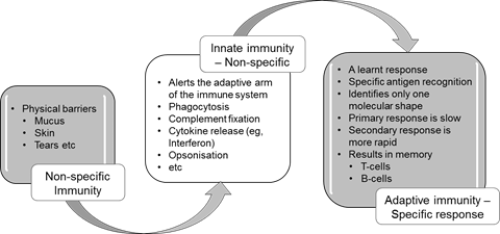
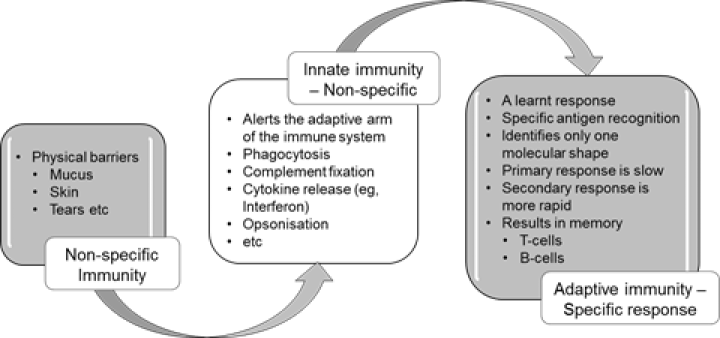
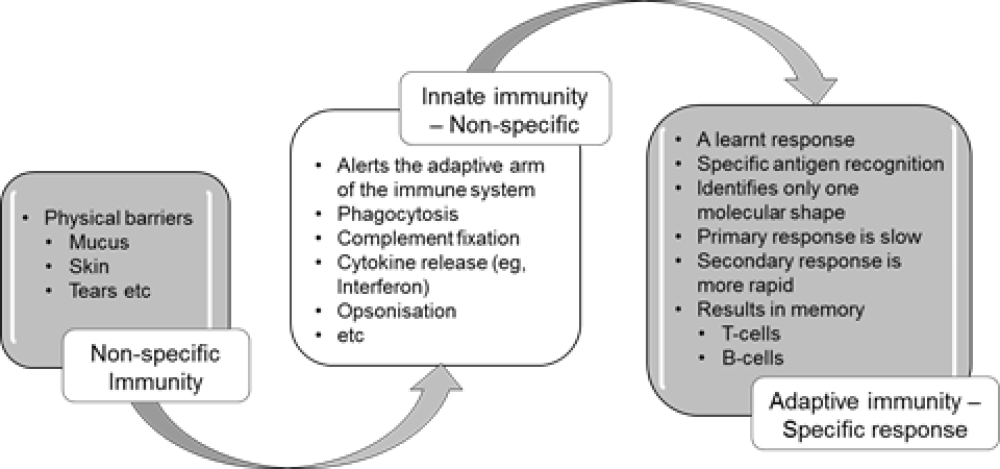
1.1.4. Acquisition of adaptive immunity
1.1.4. Acquisition of adaptive immunity
Specific immunity can be actively produced by directly responding to an antigen. This is termed adaptive or learnt immunity, in which the immune system learns to respond to specific antigens. Passive immunity is provided by transferring antibody from an immune person to temporarily protect another.
Naturally acquired immunity
Naturally acquired immunity occurs either actively by experiencing the infection or passively through the transfer of maternal antibodies from mother to fetus or infant (transplacentally or in breastmilk).
Artificially acquired immunity
‘Artificially’ acquired immunity occurs either actively through vaccination or passively through administration of immunoglobulin (IG) (see Appendix 6).
While actively acquired immunity lasts from years to life, passively acquired immunity lasts from weeks to months as the transferred antibodies decay and are not renewed.
1.1.5. Maternally derived immunity
1.1.5. Maternally derived immunity
The passive transfer of antibody from mother to fetus provides an opportunity to provide protection to the neonate against several diseases before they are old enough to be vaccinated themselves. Maternal vaccination boosts the immunity of the mother, inducing high levels of maternal antibody. This antibody is actively transported across the placenta to concentrate at protective levels by birth (in term infants).
Important diseases that maternal vaccination is effective at preventing include neonatal tetanus, influenza and pertussis in the infant for the first weeks or months of life (see section 4.1 and the relevant disease chapters).
1.1.6. Summary
1.1.6. Summary
- Successful immune responses occur following the recognition of, and appropriate response to, a foreign antigen.
- Specialised but non-specific cells, called antigen-presenting cells, take up, transport and present the vaccine antigen to antigen-specific T-cells and B-cells within the lymph nodes and spleen.
- The first wave of antibodies produced are short lived and of low affinity.
- Immune memory takes at least four months to fully develop, but the antibody and memory cells that arise are of high affinity.
- Immune memory can be boosted. This is called a secondary immune response.
- Adaptive immunity is learnt and acquired actively through disease or vaccination.
- Passive immunity is acquired through maternal transfer and administration of IG.
- Maternal vaccination offers passive protection to infants for the first weeks or months of life.
1.2. From personal protection to community (herd) immunity
By protecting individuals, vaccination can also protect the wider community. This herd immunity occurs when the vaccine coverage is high, meaning an infectious case is unlikely to encounter susceptible contacts, so transmission stops.
The whole population benefits when a vaccine prevents carriage and transmission of a human-only pathogen, such as polio virus, measles virus or Streptococcus pneumoniae, and circulation of these pathogens can be reduced and even eliminated. This phenomenon, called herd or community immunity, can prevent infections spreading and therefore protect vulnerable members of the population, such as the very young, the very old, or those with underlying conditions that increase their risk from infectious diseases (ie, the immunocompromised). These individuals may not themselves be able to receive some vaccines (eg, live vaccines) or may not mount a sufficiently effective immune response to other vaccines.
The population benefits depend on the disease itself, the nature of the vaccine and the proportion or target group of the population needed to be immunised to prevent the disease from spreading. A recent example of herd immunity in New Zealand is the significant reduction in rotavirus hospital discharge rates in children aged under 5 years following the July 2014 introduction of rotavirus vaccine for infants (see section 19.3.2).
1.2.1. Reproduction number and herd immunity threshold
1.2.1. Reproduction number and herd immunity threshold
A measure of the infectiousness of a disease is the basic reproduction number (R0). This is the number of secondary cases generated by a typical infectious individual when the rest of the population is susceptible. In other words, R0 describes the spreading potential of an infection in a population.[1] Measles is one of the most infectious diseases, with an R0 of 12–18 (Table 1.1). In other words, one person with measles is likely to infect up to 18 other susceptible people. Pertussis is similarly infectious.
If a significant proportion of the population are immune, then the chain of disease transmission is likely to be disrupted. The herd immunity threshold (H) is the proportion of immune individuals in a population that must be exceeded to prevent disease transmission. For example, to prevent measles or pertussis transmission, 92–94 percent of the population must be immune (Table 1.1).
R0 must remain above 1 for an infection to continue to exist. Once R0 drops below 1 (such as in the presence of an effective vaccination programme), the disease can be eliminated. The greater the proportion of the population that is immune to the infection, the lower the R0 will be. For example, data[2] indicates that a quadrivalent HPV vaccine programme with 70 percent coverage in young women may lead to the near disappearance of genital warts from the heterosexual population because the R0 for HPV types 6 and 11 (causing genital warts) falls to below 1 (see ‘Herd immunity and population impact’ in section 10.4.2).
Table 1.1: Approximate basic reproduction numbers (in developed countries) and implied crude herd immunity thresholds(a) for common vaccine-preventable diseases(b)
Infection |
Basic reproduction number (R0) |
Crude herd immunity threshold, H (%) |
---|---|---|
Diphtheria |
6–7 |
83–85 |
Influenzac |
1.4–4 |
30–75 |
Measles |
12–18 |
92–94 |
Mumps |
4–7 |
75–86 |
Pertussis |
5–17 |
80–94 |
Poliod |
2–20 |
50–95 |
Rubella |
6–7 |
83–85 |
Varicella |
8–10 |
Not defined |
Notes a. The herd immunity threshold (H) is calculated as 1−1/R0. b. The values given in this table are approximate: they do not properly reflect the range and diversity among populations, nor do they reflect the full immunological complexity underlying the epidemiology and persistence of these infections. c. The R0 of influenza viruses varies among subtypes. d. This is complicated by uncertainties over immunity to infection and variation related to hygiene standards. Adapted from: Fine P, Mulholland K, Scott J, et al. 2018. Community Protection. In: Plotkin S, Orenstein W, Offit P, et al (eds). Plotkin’s Vaccines (7th edition). Philadelphia, US: Elsevier. Table 77.2. |
1.2.2. Summary
1.2.2. Summary
- Not only do vaccines provide individual protection, but for many of the diseases we vaccinate against, there is also a population effect called herd/community immunity.
- Some diseases are extremely infectious and require a very high proportion of the community to be immune to prevent transmission (particularly measles and pertussis).
1.3. The importance of immunisation coverage
High immunisation coverage means more individuals are protected; it is also vital for achieving herd immunity. High coverage reduces the spread of disease to those who have not been vaccinated for medical reasons (eg, children with leukaemia while receiving treatment) or because of age (eg, infants who are too young to respond to some vaccines). High coverage also reduces the spread of disease to those who may not mount an effective immune response to vaccines because of an underlying condition (eg, those on immunosuppressive regimes).
The World Health Organization (WHO) and the New Zealand government target for immunisation coverage (since 2017/18) is for at least 95 percent of children to be fully vaccinated by age 2 years. The New Zealand target includes a marker for on-time immunisation of 95 percent by age 8 months, as well as at ages 2 years and 5 years.
This target is based on the need for:
- on-time immunisation coverage, particularly three doses of pertussis-containing vaccine for infants in the primary series and the first dose of measles vaccine at age 12 months
- achieving high herd immunity, particularly to prevent measles transmission.
For the three months ending 31 March 2020, 91 percent of New Zealand children were fully immunised by age 8 months and 92 percent were fully immunised by age 2 years. Up-to-date national and district immunisation coverage data is available at the Health New Zealand | Te Whatu Ora website.
1.4. Classification of vaccines
There are two broad categories of vaccine type: live attenuated (weakened) and non-live, which includes inactivated or whole killed, subunit and nucleic acid vaccines. Examples of the different types of vaccines are summarised in Table 1.2.
Table 1.2: Classification of vaccines, with examples
Table 1.2: Classification of vaccines, with examples
Live attenuated |
Non-live
Inactivated or whole killed |
Non-live Subunit |
Non-live Nucleic acid |
Non-live Non-replicating viral vector |
---|---|---|---|---|
Measles Mumps Rubella Varicella Rotavirus Tuberculosis (BCG) Zoster |
Poliomyelitis (IPV) Hepatitis A Some influenza vaccines |
Toxoid:
Polysaccharide:
Conjugate:
Recombinant:
Other subunit:
|
COVID-19 |
COVID-19 |
Note: Travel vaccines have been omitted from the above table. |
1.4.1. Live attenuated vaccines
1.4.1. Live attenuated vaccines
Live vaccines contain pathogens, usually viruses, which have been weakened (attenuated) so that they are able to replicate enough to induce an immune response but not cause disease. Immunity from live vaccines is usually very long-lived. The live vaccines on the National Immunisation Schedule are MMR, varicella, rotavirus and herpes zoster vaccines.
1.4.2. Non-live vaccines: Whole killed and inactivated vaccines
1.4.2. Non-live vaccines: Whole killed and inactivated vaccines
Killed vaccines contain whole bacteria that have been killed. The whole-cell pertussis vaccine is an example of a killed vaccine. There are no killed vaccines on the Schedule.
Inactivated vaccines contain viruses that have been inactivated in some way, such as splitting, so they are unable to replicate or cause disease. Examples of inactivated vaccines are influenza, hepatitis A and polio vaccines.
1.4.3. Non-live vaccines: Subunit vaccines
1.4.3. Non-live vaccines: Subunit vaccines
Subunit vaccines contain microbial fragments or particles that can induce an immune response which protects against disease. These are produced using a range of methods including recombinant engineering, detoxification processes and splitting and purification.
Toxoid vaccines
In some bacterial infections (eg, diphtheria and tetanus), the clinical manifestations of disease are caused not by the bacteria themselves but by the toxins they secrete. Toxoid vaccines are produced by harvesting a toxin and altering it chemically (usually with formaldehyde) to convert the toxin to a toxoid. The toxoid is then purified. Toxoid vaccines induce antibodies that neutralise the harmful exotoxins released from these bacteria.
Recombinant vaccines
Recombinant vaccines, such as those used against COVID-19 (rCV), hepatitis B virus (HBV) and human papillomavirus (HPV), are made using a gene from the (disease-causing) pathogen. The gene is inserted into a cell system capable of producing large amounts of the protein of interest. The protein produced can generate a protective immune response. For example, the gene for the hepatitis B surface antigen (HBsAg) is inserted into yeast cells, which replicate and produce large amounts of HBsAg. This antigen is purified and used to make vaccine. The advantage of this approach is that it results is a very pure vaccine that is efficient to produce.
Polysaccharide and conjugate vaccines
Polysaccharides are strings of sugars. Some bacteria, such as Streptococcus pneumoniae and Neisseria meningitidis, have large amounts of polysaccharide on their surface, which encapsulate the bacteria. The polysaccharide capsules protect the bacteria from the host’s immune system and can make the bacteria more virulent. Historically, it has been difficult to stimulate an effective immune response to these polysaccharide capsules using vaccines, particularly in children aged under 2 years.
First-generation capsular polysaccharide vaccines contained antigens isolated from the different polysaccharide capsules (eg, 23PPV, see chapter 16). Polysaccharide vaccines are poorly immunogenic, and they only induce a primary immune response. They produce low affinity antibodies (which do not bind well to the antigen) and, because they do not elicit T-cell responses, immune memory is not strong. Multiple priming doses (even a single dose) can cause hyporesponsiveness in both children and adults to further doses. There is also concern that repeated doses could result in ‘clonal deletion’ where the specific B-cell pool becomes depleted due to successive primary responses.
Polysaccharide conjugate vaccines (eg, Hib-PRP, PCV13 and MenACWY) contain carrier proteins that are chemically attached to the polysaccharide antigens. Attaching relatively non-immunogenic polysaccharides to the highly immunogenic carrier proteins results in activation of a T-cell response; in this way, conjugate vaccines induce both high-affinity antibodies against the polysaccharide antigens, and immune memory, and can be used in infants.
Examples of carrier proteins and vaccines that use them are:
- tetanus toxoid, used in Haemophilus influenzae type B vaccine (Hib-PRP-T; Hiberix) and meningococcal ACWY (MenACWY; MenQuadfi)
- a non-toxic recombinant variant of diphtheria toxin (CRM197), used in the 13-valent pneumococcal conjugate vaccine (PCV13; Prevenar 13)
These conjugate vaccines are limited by the number of polysaccharides that can be covalently linked to the carrier molecule, so there is still a role for polysaccharide vaccines to broaden the number of serotypes recognised. For example, PCV13 has 13 serotypes, compared to 23PPV with 23 serotypes. Polysaccharide vaccines are expected to be phased out eventually with improvements to conjugate vaccine technology and other vaccine technologies.
Principles and implications for using polysaccharide and conjugate vaccines
- Because of their improved immune response, where possible use polysaccharide-protein conjugate vaccines in preference to polysaccharide-only vaccines.
- To ensure broad protection against disease, use a conjugate vaccine to prime the immune system before using the polysaccharide vaccine to increase the number of serotypes recognised. For example, high-risk children are primed with PCV13 then boosted with 23PPV (see section 16.5.2).
- To avoid or minimise hyporesponsiveness, individuals should have a maximum of three lifetime doses of polysaccharide vaccine.
- Children aged under 2 years should not receive polysaccharide vaccines as they are likely to be ineffective in young children.
1.4.4. Non-live vaccines: Nucleic acid vaccines
1.4.4. Non-live vaccines: Nucleic acid vaccines
Recent developments in vaccine technology have allowed the use of messenger ribonucleic acid (mRNA) to deliver the genetic code to our dendritic cells make specific viral proteins. Since mRNA is easily destroyed by ubiquitous ribonuclease enzymes, it is protected inside a lipid nanoparticle that also facilitates uptake by the dendritic cells. Inside the dendritic cell, ribosomes and vaccine mRNA generate the viral protein which is then presented to the T and B cells in the lymph nodes. For example, the mRNA COVID-19 vaccine (mRNA-CV) provides the instructions to make the SARS-CoV-2 virus spike protein and induces an effective humoral and cellular immune responses against this virus.
1.4.5. Non-live vaccines: Non-replicating viral vector vaccines
1.4.5. Non-live vaccines: Non-replicating viral vector vaccines
Another recent development in vaccine design is the use of adenoviruses as a vector to deliver the instructions to human cells to make antigens. The adenovirus is genetically modified to be unable to replicate (non-replicating or replication deficient), and as such are non-live vaccines, but can deliver its double-stranded DNA into the cell’s nucleus as would normally occur during an adenovirus infection. The viral DNA contains a transgene, a portion that codes for the target antigen protein. Only this portion of the viral DNA can be expressed, thus preventing the vector from reproducing. The presence of the adenovirus triggers an immune response killing the infected cell and thereby releasing the new protein (antigen) to activate a specific immune response. For example, ChAdOx1 vector COVID-19 vaccine (ChAd-CV) uses a chimpanzee adenovirus and the Ad26 vector COVID-19 vaccine (Ad26-CV) uses a human adenovirus (Ad26) to produce SARS-CoV-2 spike proteins.
1.4.6. Summary
1.4.6. Summary
- Vaccines introduce antigens to the immune system in the form of live and non-live vaccines.
- Non-live vaccines include killed/ inactivated, subunit or nucleic acid vaccines.
- Polysaccharide vaccines do not induce immune memory and have been associated with hyporesponsiveness to later doses. Polysaccharide conjugate vaccines overcome these problems.
- Nucleic acid vaccines and viral vector vaccines provide the instructions for our own cells to make the target antigen.
1.5. Vaccine ingredients
In addition to the antigen, a vaccine may contain a range of other substances; for example, an immune enhancer (adjuvant) and/or a preservative. Traces of residual components from the manufacturing process may also be present in the vaccine. For further information on vaccine content, see the vaccine sections within the disease chapters of this Handbook.
1.5.1. Adjuvants
1.5.1. Adjuvants
Adjuvants are substances that enhance the immune response to an antigen through a range of mechanisms, including improving the delivery of the antigen to the innate immune system and to the lymphoid organs. Use of adjuvants also means that less antigen, which can be difficult to produce, is needed (this is called antigen sparing).
Adjuvants licensed for human use include aluminium salts (eg, aluminium hydroxide and aluminium phosphate), oil-in-water emulsions (MF59, Seqirus; AS03, GSK), saponin-based liposomal suspension (AS01B, GSK; Matrix-M, Novavax) and a bacterial endotoxin (AS04, GSK). Most non-live vaccines require an adjuvant, and most vaccines still use aluminium adjuvants. The amount of aluminium contained in a vaccine is very small compared with that present in our daily intake from food and water, including breastmilk.
1.5.2. Preservatives
1.5.2. Preservatives
Preservatives prevent the contamination of vaccines, particularly in multi-dose vials. 2‑phenoxyethanol is an example of a preservative used in some vaccines. It is also used in many cosmetics and baby care products. Many vaccines do not contain a preservative. Mercury-based preservatives (thiomersal/thimerosal) are not used in vaccines on the New Zealand National Immunisation Schedule, and multi-dose vials are not used for Schedule vaccinations.
1.5.3. Stabilisers
1.5.3. Stabilisers
Stabilisers protect the vaccine from adverse conditions (such as exposure to heat), inhibit chemical reactions and prevent components from separating. Examples include sucrose, lactose, albumin, gelatin, glycine and monosodium glutamate (MSG).
1.5.4. Surfactants/emulsifiers
1.5.4. Surfactants/emulsifiers
These are wetting agents that alter the surface tension of a liquid, like a detergent does. Surfactants assist particles to remain suspended in liquid, preventing settling and clumping. One commonly used surfactant is polysorbate 80, made from sorbitol (sugar alcohol) and oleic acid (an omega fatty acid). It is also commonly used in foods such as ice-cream.
1.5.5. Residuals
1.5.5. Residuals
Residuals are traces of substances that remain in the vaccine as an inevitable consequence of the manufacturing process. Regulatory bodies vary as to which trace substances must be specified. Residuals may include virus-inactivating agents (such as formaldehyde), antibiotics and other substances used in the manufacturing process, such as ovalbumin (an egg protein) and gelatin.
1.6. Safety monitoring of vaccines in New Zealand
1.6.1. The approval of vaccines for use in New Zealand
1.6.1. The approval of vaccines for use in New Zealand
Vaccines, like all medicines, have benefits and risks of harm. Before a medicine or vaccine is approved for use, it must be tested in a series of clinical trials to determine its immunogenicity, efficacy and safety profile. The data from these trials is assessed and scrutinised by regulatory authorities, such as Medsafe in New Zealand, the European Medicines Agency and the Food and Drug Administration in the US, before the medicine or vaccine is approved for use.
Known information about each medicine and vaccine is published for health professionals in a manufacturer’s data sheet, available on the Medsafe website. Consumer medicine information is usually also published.
Once the vaccine is used widely (ie, outside of the clinical trials), more information is collected on its safety profile and effectiveness. Some adverse reactions are rare and may not be seen until a very large number (thousands or even millions) of people have received the medicine or vaccine. This is one of the reasons why it is important to monitor all medicines and vaccines after they have been approved (registered). Note that some vaccines that are approved for use by Medsafe may not have been made available for distribution by the manufacturer or supplier.
Most countries (including New Zealand) have a safety monitoring system, which includes a voluntary spontaneous reporting scheme, to help identify any possible safety concerns. These reporting systems feed into the WHO Collaborating Centre for International Drug Monitoring, called the Uppsala Monitoring Centre in Sweden. This means that international data, often covering millions of doses, is available for Medsafe, which is the medicines regulator responsible for monitoring information to ensure that approved vaccines remain acceptably safe for use in New Zealand. Vaccine safety is never reviewed in isolation from the expected benefits of the vaccine; it is always looked at in terms of the risk–benefit balance.
In addition, the WHO plays an important role in monitoring vaccine safety through its Strategic Advisory Group of Experts on Immunization and the Global Advisory Committee on Vaccine Safety.
1.6.2. The New Zealand spontaneous reporting scheme
1.6.2. The New Zealand spontaneous reporting scheme
Two terms are used to describe spontaneous reports in the context of vaccination. Adverse events are undesirable events experienced by a person, which may or may not be causally associated with the vaccine. Adverse reactions are undesirable effects resulting from medicines or vaccines (ie, they are causally associated).
Spontaneous reports are case reports of adverse events that people have experienced while or after taking a medicine or having a vaccine. Medsafe contracts the collection, review and analysis of this information to the New Zealand Pharmacovigilance Centre at the University of Otago in Dunedin.
Health care professionals and consumers are encouraged to report adverse events following immunisation (AEFIs) to the Centre for Adverse Reactions Monitoring (CARM), which is part of the New Zealand Pharmacovigilance Centre. Pharmaceutical companies also submit adverse event reports.
Further information about suspected adverse reactions (and events following immunisation) reported in New Zealand can be found in the Suspected Medicine Adverse Reaction Search on the Medsafe website. See below for details about how to report to CARM and what information should be reported.
1.6.3. AEFI reporting process – notifying CARM
1.6.3. AEFI reporting process – notifying CARM
When obtaining consent for immunisation, vaccinators should also seek consent to report any adverse events that may occur, because AEFI reporting is considered part of immunisation programme quality control monitoring and public safety.
How to report to CARM
Adverse events may be reported to CARM through:
- the electronic adverse reaction reporting tool available in practice management software programmes
- online reporting
For support with completing a CARM report please call: 0800 400569.
To submit a clinical document or if enquiring about a submitted report, please send an email to: CARMreport@health.govt.nz
In terms of guidance, the sort of information the reporting form generally requires is a patient identifier (gender, age, initial), a medicine, a reaction and the reporter’s contact details.
This information can also be accessed from the Medsafe website (revised 28 August 2023).
What should be reported?
Health professionals/vaccinators should report:
- all serious suspected AEFI and other reactions of clinical concern to established vaccines, such as those described in Table 1.3 below. The AEFIs should be reported regardless of whether they consider the event to have been caused by the vaccination, and they should still be reported even if the effect is well recognised
- all suspected adverse reactions (including minor reactions) to newly introduced vaccines, or those being used for new indications or being delivered by a different route.
Individuals or parents/guardians should be encouraged to notify vaccinators of any AEFI that they consider may have been caused by the vaccination. Alternatively, individuals or parents/guardians may wish to notify CARM themselves, or they can contact their general practice or the Immunisation Advisory Centre (IMAC) on 0800 IMMUNE/0800 466 863 to help with notification.
If in doubt, report it.
Table 1.3: Examples of AEFIs to be reported
Timeframe |
Event |
---|---|
All vaccines |
|
Within 24 hours of vaccination |
Anaphylactic reaction (acute hypersensitivity reaction) |
Within 5 days of vaccination |
Severe local reaction |
Within 12 days of vaccination |
Seizures, including febrile seizures |
Within 3 months of vaccination |
Acute flaccid paralysis* (AFP), including Guillain–Barré syndrome (GBS) |
Between 1 and 12 months after BCG vaccination |
Lymphadenitis |
No time limit |
Intussusception after rotavirus vaccine |
Newly introduced vaccines, or those with new indications or being delivered by a different route |
|
No time limit |
All suspected adverse reactions |
* AFP in children is also monitored by the New Zealand Paediatric Surveillance Unit as part of polio eradication surveillance (see Chapter 17). |
Seriousness of AEFIs
Reports of suspected adverse reactions or AEFIs can be categorised as serious or non-serious. This categorisation system is a tool used to try and prioritise safety concerns. It is not a reflection of the importance of the events to the consumer or their health care professional. Because a report is defined as serious based on what is reported, it is possible to have both serious and non-serious cases reporting the same type of event; for example, headache.
International convention defines the seriousness of reports based on the outcome or nature of the reported event as documented in the report, irrespective of whether there is any association to the medicine or vaccine.
Serious events are based on the following international criteria:
- hospitalisation (or prolonged hospitalisation) of the patient
- life-threatening event
- persisting disability of the patient
- intervention required to prevent permanent impairment
- congenital anomaly
- death of the patient.
CARM assessment of causality
The WHO recommends that individual reports of adverse reactions to vaccines are assessed for causality. This assessment is a tool used to help detect new safety concerns; it is not a determination of whether a vaccine caused an adverse reaction.
The person reporting the event will receive a letter of response from CARM commenting on the adverse effect, the causal relationship and the number of other similar events, and advice about future use of the vaccine in the individual. Also, where applicable, CARM will provide a validated AEFI code to the AIR.
The information provided by CARM:
- needs to be communicated to the individual and parent/guardian (if applicable)
- must be entered in the medical notes
- will help to identify those individuals who should receive follow-up vaccination in a controlled environment, such as a hospital.
1.6.4. What does Medsafe do with this information?
1.6.4. What does Medsafe do with this information?
Medsafe and CARM analyse spontaneous reports in conjunction with other information to determine whether there are any new potential safety signals. Medsafe seeks the advice of independent experts through the Medicines Adverse Reactions Committee or may form working groups of experts to provide advice. Medsafe works closely with other regulatory authorities from around the world.
Medsafe undertakes a risk–benefit assessment of safety signals to decide if action is required. Further information on risk–benefit assessment is provided on the Medsafe website.
Most safety signals are not supported by any additional information, and no action is taken, although Medsafe may continue to monitor the issue closely. A small number of possible safety signals are confirmed as real. In these cases, Medsafe has several regulatory actions it can take, including withdrawing the product.
In New Zealand, it is less likely that any rare new side-effects to vaccines will be detected because of the small number of people immunised compared to other countries. Therefore, Medsafe uses international data available from the WHO, other regulators and pharmaceutical companies to help assess any reports of rare events following immunisation and to determine if they may be new events linked to immunisation.
1.6.5. Advantages and limitations of spontaneous reports
1.6.5. Advantages and limitations of spontaneous reports
Spontaneous reports have been shown to be a very simple way of identifying potential or possible safety signals with medicines, and over 90 countries have a spontaneous reporting system. They can be used to monitor the safety of medicines in real-life use over the lifetime of the medicine, and for all types of people.
The limitations of using spontaneous reports include under-reporting, a lack of reliable information on the extent of use of the medicine and wide variations in the clinical details provided about the event and the history of the patient. Spontaneous reports are heavily subject to reporting bias, such as media or other attention on an issue. They are also not very effective at detecting adverse reactions that occur a long time after starting the medicine.
For these reasons, such reports are only used to identify safety signals. These signals require further formal epidemiological study before they can be validated or discounted. Information obtained from spontaneous reports needs to be interpreted with caution.
Understanding vaccine safety and spontaneous reporting
Spontaneous report patterns can be variable, and they depend on many factors. Summaries of reported events following immunisation are not lists of known or proven adverse reactions to vaccines. They cannot be used to determine the frequency of adverse reactions to vaccines in the whole population, and they cannot be used to directly compare the relative safety of vaccines. They must not be interpreted and used as such.
Health care professionals and consumers are encouraged to report any suspicions that an event they have experienced may have been caused by vaccination. Therefore, reports sent to CARM may be:
- real adverse reactions to the vaccine
- anxiety or nervousness about needles or the process of vaccination
- coincidental events that would have occurred anyway.
With any vaccine, the adverse events that are generally reported include:
- injection-site reactions
- well-recognised events, such as headaches, dizziness, muscle aches, mild fever and tiredness
- mild allergic reactions, such as mild rashes and itching
- rare but serious allergic reactions, called anaphylaxis, which can occur in response to any medicine or vaccine and some foods – health care professionals giving vaccines are trained to recognise the symptoms of serious allergic reactions and promptly treat them
- immunisation stress-related responses due to fear or anticipation of the needle injection (eg, fainting or hyperventilation)
- coincidental medical conditions
- new adverse events (ie, those not already listed in the prescribing information [data sheet]).
There will always be a few coincidental events reported because vaccines are given to large sections of the population. In some cases, vaccines are specifically targeted at people with underlying medical conditions (eg, the influenza vaccine). The challenge is to be able to distinguish these coincidental ‘background’ events from those that may have been caused by the vaccine. There are a range of research methods for assessing the risk of an event after a vaccine compared with the risk with no vaccine exposure.
The time between immunisation and an event can be important in determining whether the event was coincidental. Most reactions to vaccines occur within a very short time of immunisation, usually within days.
Another important approach taken when assessing vaccine safety is comparing the number of reports for a specific event with the expected background rate for that event. When doing this, it is important to ensure that definite diagnoses of the events reported were made and to adjust the background rate for any differences in population groups and seasonal variations.[3]
References
References
References
- Fine P, Mulholland K, Scott J, et al. 2018. Community Protection, in Plotkin's Vaccines (7th edition), Plotkin S, Orenstein W, Offit P, et al. (eds). Elsevier: Philadelphia, US.
- Read TR, Hocking JS, Chen MY, et al. The near disappearance of genital warts in young women 4 years after commencing a national human papillomavirus (HPV) vaccination programme. Sexually Transmitted Infections, 2011. 87(7): 544–7.
- Sexton K, McNicholas A, Galloway Y, et al. Henoch-Schönlein purpura and meningococcal B vaccination. Archives of Disease in Childhood, 2009. 94(3): 224–6.